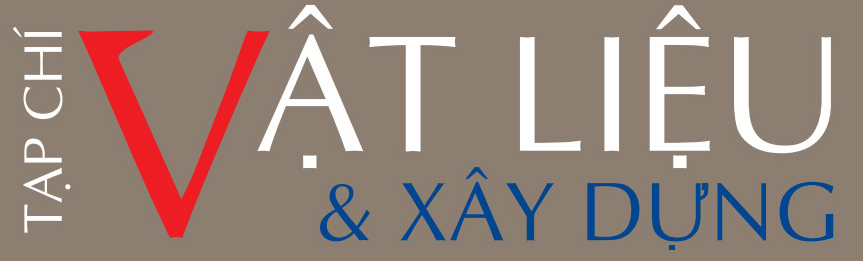
ISSN:
Website: www.jomc.vn
Nghiên cứu phát triển xi măng sinh học có nguồn gốc từ thực vật nhằm giảm phát thải CO2 trong lĩnh vực xây dựng
Tóm tắt
Nghiên cứu này sử dụng phương pháp tạo kết tủa CaCO3 từ Enzyme Urease (Enzyme Induced Carbonate Precipitation - EICP) để sản xuất xi măng sinh học. Enzyme thô (chi phí thấp, thân thiện với môi trường) được chiết xuất từ hạt đậu nành (soybeans) có hoạt tính Urease cao thích hợp cho sản xuất xi măng sinh học. Hoạt tính của enzyme được xác định đồng thời với sự thay đổi pH và độ dẫn điện của dung dịch khi thủy phân urea (CO(NH2)2). Kỹ thuật EICP dùng để xi măng hóa các mẫu cát hình trụ. Kết tủa tạo ra từ xi măng sinh học có khả năng kết dính các hạt cát lại với nhau vì vậy tăng cường độ của mẫu cát với các giá trị lớn nhất và nhỏ nhất ứng với cường độ nén lần lượt là 1004,2 kPa và 359,9 kPa, đồng thời chèn vào lỗ rỗng giữa các hạt làm giảm tính thấm với độ thấm ban đầu là 10-1 cm/s, Kết tủa CaCO3 được xác định bằng các phân tích vi mô sử dụng kỹ thuật nhiễu xạ tia X và kính hiển vi điện tử. Ngoài ra, nghiên cứu còn đánh giá chi phí và lợi ích môi trường về việc giảm phát thải CO2 so với xi măng Portland thông thường.
Tài liệu tham khảo
- H. Klee, E. Coles, and A. Costolloe, “The cement sustainability initiative Implementing change across a global industry,” Corp. Soc. Responsib. Environ. Manag., vol. 11, no. 2, pp. 114–120, 2004.
- C. Meyer, “The greening of the concrete industry,” Cem. Concr. Compos., vol.31, no. 8, pp. 601–605, 2009.
- E. Gartner, “Industrially interesting approaches to ‘low-CO2’ cements,” Cem. Concr. Res., vol. 34, no. 9, pp. 1489–1498, 2004.
- V. S. Whiffin, “Microbial CaCO3 precipitation for the production of biocement,” Murdoch University, Australia, 2004.
- V. Rebata-Landa and J. C. Santamarina, “Mechanical limits to microbial activity in deep sediments,” Geochemistry, Geophys. Geosystems, vol. 7, no. 11, pp. 1–12, 2006.
- D. Ran and S. Kawasaki, “Effective use of plant-derived urease in the field of geoenvironmental/geotechnical engineering,” J. Civ. Environ. Eng., vol. 6, no. 1, p. 207, 2016.
- E. Kavazanjian and N. Hamdan, “Enzyme induced carbonate precipitation (EICP) columns for ground improvement,” in Proc., ASCE IFCEE 2015, 2015, pp. 2252–2261.
- H. Yasuhara, D. Neupane, K. Hayashi, and M. Okamura, “Experiments and predictions of physical properties of sand cemented by enzymatically-induced carbonate precipitation,” Soils Found., vol. 52, no. 3, pp. 539–549, 2012.
- D. Neupane, H. Yasuhara, N. Kinoshita, and T. Unno, “Applicability of enzymatic calcium carbonate precipitation as a soil-strengthening technique,” ASCE J. Geotech. Geoenvironmental Eng., vol. 139, no. December, pp. 2201–2211, 2013.
- N. Hamdan and E. Kavazanjian, “Enzyme-induced carbonate mineral precipitation for fugitive dust control,” Géotechnique, vol. 66, no. 7, pp. 546–555, 2016.
- T. Hoang, J. Alleman, B. Cetin, K. Ikuma, and S.-G. Choi, “Sand and silty-sand soil stabilization using bacterial enzyme induced calcite precipitation (BEICP),” Can. Geotech. J., vol. 56, no. 6, pp. 808–822, 2019.
- R. A. N. Dilrukshi, J. Watanabe, and S. Kawasaki, “Strengthening of sand cemented with Calcium Phosphate Compounds using Plant-derived Urease,” Int. J. Geomate, vol. 11, no. 25, pp. 2461–2467, 2016.
- ASTM. 2010. Standard practice for classification of soils for engineering purposes (Unified Soil Classification System). ASTM D2487-10. ASTM.
- ASTM. 2006. Standard test method for permeability of granular soils (constant head). ASTM D2434-68. ASTM.
- Cheng, L., Cord-Ruwisch, R., and Shahin, M.A. 2013. Cementation of sand soil by microbially induced calcite precipitation at various degrees of saturation. Canadian Geotechnical Journal, 50(1): 81–90.
- ASTM. 1996. Standard test method for unconfined compressive strength index of chemical grouted soil. ASTM D4219-08. ASTM.
- Feng, K., and Montoya, B.M. 2015. Influence of confinement and cementation level on the behavior of microbial-induced calcite precipitated sands under monotonic drained loading. Journal of Geotechnical and Geoenvironmental Engineering, 2: 1–9.
- Wani, A.A.; Sogi, D.S.; Singh, P.; Wani, I.A.; Shivhare, U.S. Characterisation and functional properties of watermelon (Citrullus lanatus) seed proteins. J. Sci. of Food Agric. 2011, 91, 113–121.
- Abas Wani, A.; Sogi, D.S.; Grover, L.; Saxena, D.C. Effect of Temperature, Alkali Concentration, Mixing Time and Meal/Solvent Ratio on the Extraction of Watermelon Seed Proteins—A Response Surface Approach. Biosyst. Eng. 2006, 94, 67–73.
- Whiffin, V. S. (2004). “Microbial CaCO3 precipitation for the production of biocement.” Ph.D. dissertation, Murdoch University, Australia.
- Imran, M. Al, Nakashima, K., and Kawasaki, S. (2021). “Bio-mediated soil improvement using plant derived enzyme in addition to magnesium ion.” Crystals, 11(5).
- Almajed A., Tirkolaei H.K. and Kavazanjian E., Baseline investigation on enzyme-induced calcium carbonate precipitation., Journal of Geotechnology and Geoenvironmental Eng., Vol. 144, Issue 11, 2018, pp. 1-11.
- Al-Thawadi S., Cord-Ruwisch R., Calcium Carbonate Crystals Formation by Ureolytic Bacteria Isolated from Australian Soil and Sludge. J. Adv. Sci. Eng. Res., Vol. 2, 2012, pp. 12-26.
- Nemati, M., and Voordouw, G. 2003. Modification of porous media permeability, using calcium carbonate produced enzymatically in situ. Enzyme and Microbial Technology, 33(5): 635–642.
- Al Qabany, A., and Soga, K. 2013. Effect of chemical treatment used in MICP on engineering properties of cemented soils. Géotechnique, 63(4): 331–339.
- Choi, S.-G., Wang, K., and Chu, J. 2016. Properties of biocemented, fiber reinforced sand. Construction and Building Materials, 120: 623–629.
- Yasuhara, H., Neupane, D., Hayashi, K., and Okamura, M. 2012. Experiments and predictions of physical properties of sand cemented by enzymaticallyinduced carbonate precipitation. Soils and Foundations, 52(3): 539–549.
- Van Paassen, L.A.; Ghose, R.; Van Der Linden, T.J.M.; Van Der Star, W.; Van Loosdrecht, M.C.M. Quantifying Biomediated Ground Improvement by Ureolysis: Large Scale Biogrout Experiment. J. Geotech. Geoenviron. Eng. 2010, 136, 1721–1728
- Van Der Linden, T.J.M. 2010b. Strength and deformation of biologically cemented sandstone. In Proceedings of Rock Engineering in Difficult Ground Conditions - Soft Rocks and Karst. Edited by I. Vrkljan. CRC Press, Cavtat, Croatia, pp. 405–410
- Cui, M.J., Zheng, J.J., Zhang, R.J., Lai, H.J., and Zhang, J. 2017. Influence of cementation level on the strength behaviour of bio-cemented sand. Acta Geotechnica, 12(5): 971–986. doi:10.1007/s11440-017-0574-9.
- Gomez, M.G.; Anderson, C.M.; Graddy, C.M.R.; DeJong, J.; Nelson, D.C.; Ginn, T.R. Large-Scale Comparison of Bioaugmentation and Biostimulation Approaches for Biocementation of Sands. J. Geotech. Geoenviron. Eng. 2017, 143, 04016124.
- Van Paassen, L.A. Bio-Mediated Ground Improvement: From Laboratory Experiment to Pilot Applications. Geo-Frontiers 2011 2011, 4099–4108.
- Gomez, M.G.; Martinez, B.C.; DeJong, J.; Hunt, C.E.; DeVlaming, L.A.; Major, D.W.; Dworatzek, S.M. Field-scale bio-cementation tests to improve sands. In Institution of Civil Engineers-Ground Improvement; Thomas Telford Ltd.: London, UK, 2015; Volume 168, pp. 206–216.
- Gomez, M.G.; DeJong, J.; Anderson, C.M.; Nelson, D.C.; Graddy, C.M.R. Large-Scale Bio-Cementation Improvement of Sands. Geotechnical and Structural Engineering Congress 2016 2016, 941–949.
- Nassar, M.K.; Gurung, D.; Bastani, M.; Ginn, T.; Shafei, B.; Gomez, M.; Graddy, C.M.R.; Nelson, D.C.; DeJong, J.T. Large-Scale Experiments in Microbially Induced Calcite Precipitation (MICP): Reactive Transport Model Development and Prediction. Water Resour. Res. 2018, 54, 480–500.
- Porter, H.; Mistri, A.; Mukherjee, A. Sustainable Road bases with microbial precipitation. In Institution of Civil Engineers-Construction Materials; Thomas Telford Ltd.: London, UK, 2018; Volume 171, pp. 95–108.
- Achal, V.; Mukherjee, A.; Reddy, M.S. Microbial Concrete: Way to Enhance the Durability of Building Structures. J. Mater. Civ. Eng. 2011, 23, 730–734.
- Ghosh, P.; Mandal, S.; Chattopadhyay, B.; Pal, S. Use of microorganism to improve the strength of cement mortar. Cem. Concr. Res. 2005, 35, 1980–1983.
- Achal, V.; Mukherjee, A.; Basu, P.C.; Reddy, M.S. Strain improvement of Sporosarcina pasteurii for enhanced urease and calcite production. J. Ind. Microbiol. Biotechnol. 2009, 36, 981–988.
- Piasta, W.; Zarzycki, B. The effect of cement paste volume and w/c ratio on shrinkage strain, water absorption and compressive strength of high-performance concrete. Constr. Build. Mater. 2017, 140, 395–402.
- ACI. ACI Manual of Concrete Practice 2000, Part 1: Materials and General Properties of Concrete; American Concrete Institute: Farmington Hills, MI, USA, 2000.
- Rahman, M.; Beecham, S.; Iqbal, A.; Karim, R.; Rabbi, A.T.Z. Sustainability Assessment of Using Recycled Aggregates in Concrete Block Pavements. Sustainability 2020, 12, 4313.
- Azam, A.M.; Cameron, D.A.; Rahman, M. Permanent Strain of Unsaturated Unbound Granular Materials from Construction and Demolition Waste. J. Mater. Civ. Eng. 2015, 27, 04014125.
- Azam, A.; Cameron, D.A.; Rahman, M. Model for prediction of resilient modulus incorporating matric suction for recycled unbound granular materials. Can. Geotech. J. 2013, 50, 1143–1158.
- Rahman, M.M.; Beecham, S.; McIntyre, E.; Iqbal, A. Mechanistic Design of Concrete Block Pavements. In Australian Geomechanics Society Victorian Symposium: Geotechnics and Transport. Infrastucture; King, D., Ed.; Australian Geomechanics Society: Melbourne, Australia, 2018; pp. 13–17.
- Rahman, M.M.; Beecham, S.; McIntyre, E. A new approach to the mechanistic design and analysis of concrete block pavement. In Proceedings of the 12 International Conference on Concrete Block Pavement, Seoul, Korea, 16–19 October 2018; pp. 37–41.
- Sarda, D.; Choonia, H.S.; Sarode, D.D.; Lele, S. Biocalcification by Bacillus pasteurii urease: A novel application. J. Ind. Microbiol. Biotechnol. 2009, 36, 1111–1115.
- Omoregie, A.I.; Ngu, L.H.; Ong, D.E.; Nissom, P.M. Low-cost cultivation of Sporosarcina pasteurii strain in food-grade yeast extract medium for microbially induced carbonate precipitation (MICP) application. Biocatal. Agric. Biotechnol. 2019, 17, 247–255.
- Fang, C.; He, J.; Achal, V.; Plaza, G. Tofu Wastewater as Efficient Nutritional Source in Biocementation for Improved Mechanical Strength of Cement Mortars. Geomicrobiol. J. 2019, 36, 515–521.
- Madlool, N.; Saidur, R.; Hossain, M.; Rahim, N.A. A critical review on energy use and savings in the cement industries. Renew. Sustain. Energy Rev. 2011, 15, 2042–2060.
- NRMCA. Concrete CO2 Fact Sheet; National Ready Mixed Concrete Association: Alexandria, VA, USA, 2012.
- CA. Gas. Price Trends Review; Department of Industry, Innovation and Science, Commonwealth of Australia: Canberra, Australia, 2015
- APPEA. Natural Gas–Essential for Australian Manufacturing; The Australian Petroleum Production & Exploration Association: Canberra, Australia, 2016.
- DTE. Indian Urea Plants Comparable to the Best Worldwide: Study; DownToEarth: New Delhi, India, 2019.
- CW. Emission Factors in kg CO2-Equivalent Per Unit; City of Winnipeg: Manitoba, Canada, 2012.
- Sigurdarson, J.J.; Svane, S.; Karring, H. The molecular processes of urea hydrolysis in relation to ammonia emissions from agriculture. Rev. Environ. Sci. Bio/Technol. 2018, 17, 241–258.
- Ivanov, V.; Chu, J. Applications of microorganisms to geotechnical engineering for bioclogging and biocementation of soil in situ. Rev. Environ. Sci. Bio/Technol. 2008, 7, 139–153.