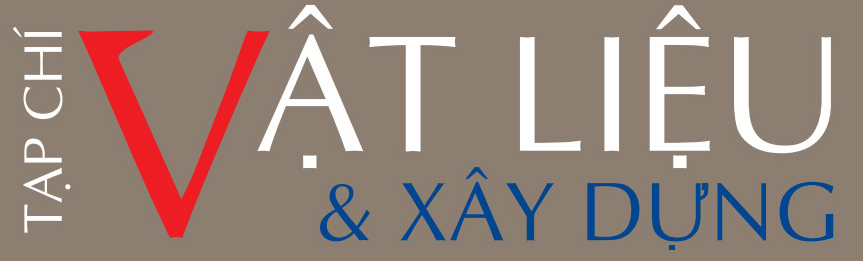
ISSN:
Website: www.jomc.vn
Nghiên cứu các mô hình mô phỏng năng lượng: phân loại, ứng dụng kỹ thuật, xu hướng nghiên cứu và phát triển
Tóm tắt
Hiện nay tốc độ đô thị hóa ở Việt Nam ngày càng tăng đã kéo theo những áp lực liên quan đến nhu cầu sử dụng năng lượng trong lĩnh vực xây dựng. Tiêu thụ năng lượng trong các công trình dân dụng và công nghiệp chiếm tỉ trọng lớn trong tổng tiêu thụ năng lượng quốc gia. Tuy nhiên, cho đến nay nhiều công trình xây dựng tại Việt Nam vẫn chưa chú trọng đúng mức việc tích hợp tính hiệu quả sử dụng năng lượng vào các khâu thiết kế, xây dựng và vận hành công trình. Việc áp dụng các mô hình mô phỏng năng lượng trong các quy trình thiết kế, xây dựng và vận hành công trình có thể góp phần làm giảm đáng kể tổng mức tiêu thụ năng lượng và giảm thiểu phát thải khí nhà kính trong lĩnh vực xây dựng. Tuy nhiên, các nghiên cứu và áp dụng thực tế các mô phỏng năng lượng công trình trong các giai đoạn thiết kế và vận hành chưa được áp dụng phổ biến ở Việt nam. Vì vậy, bài báo này đã tổng quan các nghiên cứu trên thế giới để cung cấp thông tin đầy đủ, chi tiết về các khái niệm, phân loại, ứng dụng kỹ thuật, xu hướng phát triển của mô hình, phần mềm mô phỏng năng lượng công trình trong lĩnh vực xây dựng. Nghiên cứu này là tài liệu tham khảo cho những nghiên cứu trong tương lai ở Việt Nam về ứng dụng mô phỏng năng lượng công trình ở các giai đoạn thiết kế và vận hành công trình nhằm đảm bảo tiết kiệm năng lượng, giảm thiểu phát thải cacbon mà vẫn duy trì tiện nghi môi trường.
Tài liệu tham khảo
- . J. Sousa, “Energy Simulation Software for Buildings : Review and Comparison.”
- . S. A. Klein, W. A. Beckman, and J. A. Duffie, “Trnysys - a Transient Simulation Program.,” ASHRAE Trans., vol. 82, no. pt 1, pp. 623–633, 1976.
- . T. Kalamees, “IDA ICE: the simulation tool for making the whole building energy- and HAM analysis,” IEA Annex 41 MOIST-ENG, Work. Meet. May 12-14, no. 1999, p. 6, 2004.
- . F. Nocera, A. Lo Faro, V. Costanzo, and C. Raciti, “Daylight Performance of Classrooms in a Mediterranean School Heritage Building,” Sustainability, vol. 10, no. 10, p. 3705, Oct. 2018.
- . C. Tam, Y. Zhao, Z. Liao, and L. Zhao, “Mitigation Strategies for Overheating and High Carbon Dioxide Concentration within Institutional Buildings: A Case Study in Toronto, Canada,” Buildings, vol. 10, no. 7, p. 124, Jul. 2020.
- . I. García Kerdan, R. Raslan, and P. Ruyssevelt, “An exergy-based multi-objective optimisation model for energy retrofit strategies in non-domestic buildings,” Energy, vol. 117, pp. 506–522, Dec. 2016.
- . B. J. Futrell, E. C. Ozelkan, and D. Brentrup, “Bi-objective optimization of building enclosure design for thermal and lighting performance,” Build. Environ., vol. 92, pp. 591–602, Oct. 2015.
- . A. Garnier, J. Eynard, M. Caussanel, and S. Grieu, “Predictive control of multizone heating, ventilation and air-conditioning systems in non-residential buildings,” Appl. Soft Comput., vol. 37, pp. 847–862, Dec. 2015.
- . X. Li and J. Wen, “Review of building energy modeling for control and operation,” Renew. Sustain. Energy Rev., vol. 37, pp. 517–537, 2014.
- . R. K. Strand et al., “EnergyPlus : A New-Generation Energy Analysis and Load Calculation Engine for Building Design Introduction : What is EnergyPlus ?,” ACSA Technol. Conf., vol. 2, no. April 2014, 2000.
- . University of Strathclyde, “The ESP-r System for Building Energy Simulation,” 2002.
- . J. Ma, J. Qin, T. Salsbury, and P. Xu, “Demand reduction in building energy systems based on economic model predictive control,” Chem. Eng. Sci., vol. 67, no. 1, pp. 92–100, Jan. 2012.
- . J. W. Moon and J.-J. Kim, “ANN-based thermal control models for residential buildings,” Build. Environ., vol. 45, no. 7, pp. 1612–1625, Jul. 2010.
- . R. Yokoyama, T. Wakui, and R. Satake, “Prediction of energy demands using neural network with model identification by global optimization,” Energy Convers. Manag., vol. 50, no. 2, pp. 319–327, Feb. 2009.
- . B. J. Urban, “The MIT Design Advisor: simple and rapid energy simulation of early-stage building designs,” Massachusetts Inst. Technol., no. September 2008, 2007.
- . S. Luziani and B. Paramita, “Autodesk Green Building Studio an Energy Simulation Analysis in the Design Process,” KnE Soc. Sci., vol. 2019, pp. 735–749, 2019.
- . S. Lamichhane, “Graduate Theses, Dissertations, and Problem Reports An eQUEST Based Building Energy Modeling Analysis for Energy An eQUEST Based Building Energy Modeling Analysis for Energy Efficiency of Buildings Efficiency of Buildings,” 2021.
- . H. A. Wasilowski and C. F. Reinhart, “Energy Simulation of an Existing Building Using Customized Internal Load Schedules and Weather Data as Opposed to Default Assumptions,” no. July, pp. 1252–1259, 2009.
- . A. Zachariah, P. Rao, B. Corn, and D. Davison, “Design,” 2022.
- . O. Ahmed and T. Al-Zubaydi, “Building Models Design And Energy Simulation With Google Sketchup And,” J. Adv. Sci. Eng. Res., vol. 3, no. 4, pp. 318–333, 2013.
- . F. Del and A. Gonzalo, “Assessment of Building Energy Simulation Tools to Predict Heating and Cooling Energy Consumption at Early Design Stages,” 2023.
- . Y. Pan et al., “Advances in Applied Energy Building energy simulation and its application for building performance optimization : A review of methods, tools, and case studies,” Adv. Appl. Energy, vol. 10, no. March, p. 100135, 2023.
- . I. Loche, C. Bleil de Souza, A. B. Spaeth, and L. O. Neves, “Decision-making pathways to daylight efficiency for office buildings with balconies in the tropics,” J. Build. Eng., vol. 43, p. 102596, Nov. 2021.
- . M. Gercek and Z. Durmuş Arsan, “Energy and environmental performance based decision support process for early design stages of residential buildings under climate change,” Sustain. Cities Soc., vol. 48, p. 101580, Jul. 2019.
- . J. Yao, J. Zhong, and N. Yang, “Indoor air quality test and air distribution CFD simulation in hospital consulting room,” Int. J. Low-Carbon Technol., vol. 17, pp. 33–37, Feb. 2022.
- . S. Lu, B. Lin, and C. Wang, “Investigation on the potential of improving daylight efficiency of office buildings by curved facade optimization,” Build. Simul., vol. 13, no. 2, pp. 287–303, Apr. 2020.
- . D. Yan, J. Xia, W. Tang, F. Song, X. Zhang, and Y. Jiang, “DeST — An integrated building simulation toolkit Part I: Fundamentals,” Build. Simul., vol. 1, no. 2, pp. 95–110, 2008.
- . M. Wetter, “A modelica-based model library for building energy and control systems,” IBPSA 2009 - Int. Build. Perform. Simul. Assoc. 2009, no. June, pp. 652–659, 2009.
- . S. Huang, W. Zuo, and M. D. Sohn, “Improved cooling tower control of legacy chiller plants by optimizing the condenser water set point,” Build. Environ., vol. 111, pp. 33–46, Jan. 2017.
- . J. Gomez-Romero et al., “A Probabilistic Algorithm for Predictive Control With Full-Complexity Models in Non-Residential Buildings,” IEEE Access, vol. 7, pp. 38748–38765, 2019.
- . F. Souayfane, R. M. Lima, H. Dahrouj, and O. Knio, “A weather-clustering and energy-thermal comfort optimization methodology for indoor cooling in subtropical desert climates,” J. Build. Eng., vol. 51, p. 104327, Jul. 2022.
- . S. Papadopoulos, C. E. Kontokosta, A. Vlachokostas, and E. Azar, “Rethinking HVAC temperature setpoints in commercial buildings: The potential for zero-cost energy savings and comfort improvement in different climates,” Build. Environ., vol. 155, pp. 350–359, May 2019.
- . C. Fan et al., “Open-source Modelica models for the control performance simulation of chiller plants with water-side economizer,” Appl. Energy, vol. 299, p. 117337, Oct. 2021.